1 Body Shape, Behavior and Propagation
1.1 Introduction to Males
In addition to hermaphrodites (5AA; XX), C. elegans produces a male sex (5AA; X0). Males differ from hermaphrodites in their gross morphology, many aspects of their anatomy and expression of certain behaviors. Most sex-specific differences are established after hatching, over the course of larval development. Under the stereomicroscope, the adult male can be distinguished from the hermaphrodite by its slim body, clear (white) ventral gonad and distinctive tail, which bears a copulatory apparatus (MaleIntroFIG 1). Adult males are smaller than aged-matched hermaphrodites, despite the fact that they are composed of more somatic nuclei. The greater girth and length of hermaphrodites appears to be due, in part, to their voluminous gonad although other tissues, such as the hypodermis, may contribute (Hodgkin, 1988). The cell lineage of the male, from egg to adult, has been determined and, like the hermaphrodite, appears to be largely invariant between individuals (Sulston and Horvitz, 1977; Sulston et al., 1980; Sulston et al., 1983). In contrast to the hermaphrodite, earlier descriptions of the connectivity of the male nervous system were only partial (Sulston et al., 1980). Reconstruction of the whole male nervous system from serial EM sections as per the hermaphrodite (White et al., 1986; Hall and Russell, 1991), is currently under way (The Male Wiring Project). Data emerging from this project is being incorporated into the male handbook and Individual neurons pages.

MaleIntroFIG 1: Light microscopy images showing male and hermaphrodite anatomy. A. Male. Inset shows a magnified view of the tail region by Nomarski DIC. B. Hermaphrodite.
Similar to hermaphrodites, (esp younger) males survive being kept frozen for long periods of time. C. elegans male larvae also switch to the facultative diapause stage called the dauer larva which can survive four to eight times the normal 3-week life span (Cassada and Russell, 1975).
Besides the large repertoire of behavior seen in hermaphrodites (foraging; feeding; defecation; dauer larva formation; sensory responses to touch, smell, taste, and temperature, social behavior, and learning and memory ) males display some other complex behaviors like male mating (Rankin, 2002; de Bono, 2003).
1.2 Male Body Shape
At hatching male larvae have the same cylindrical body form as hermaphrodites. However, as they progress through the larval stages and their sexual organs develop, the shape of their posterior changes (Sulston and Horvitz, 1977; Sulston et al., 1980; Nguyen et al., 1999). The pointed (leptoderan) tail tip retracts generating a blunt-ended (peloderan) tail. The male's copulatory apparatus, which consists of several structures, is also established in the tail. A lateral extension of the cuticle, called the fan, extends from the tail and holds nine bilateral pairs of sensory rays (MaleIntroFIG 1A, 2A and 2B). On the ventral surface of the tail, on either side of the cloaca (male anus), are the hook sensillum and postcloacal sensilla (PCS) (MaleIntroFIG 2B). The cloaca is connected internally to a chamber called the proctodeum (a modified rectum), which in turn is linked to the intestine and the gonad (MaleIntroFIG 1A). The proctodeum houses prong-like structures called the spicules, two sensilla covered in a hard, sclerotic cuticle. The rays, hook, PCS and spicules have been shown to have specific roles in guiding the execution of male mating behavior (MaleIntroFIG 3 and MaleIntroMOVIE 1; Liu and Sternberg, 1995) (see also Male Neuronal Support Cells: Rays, Hook, Spicules and Post-cloacal Sensilla.
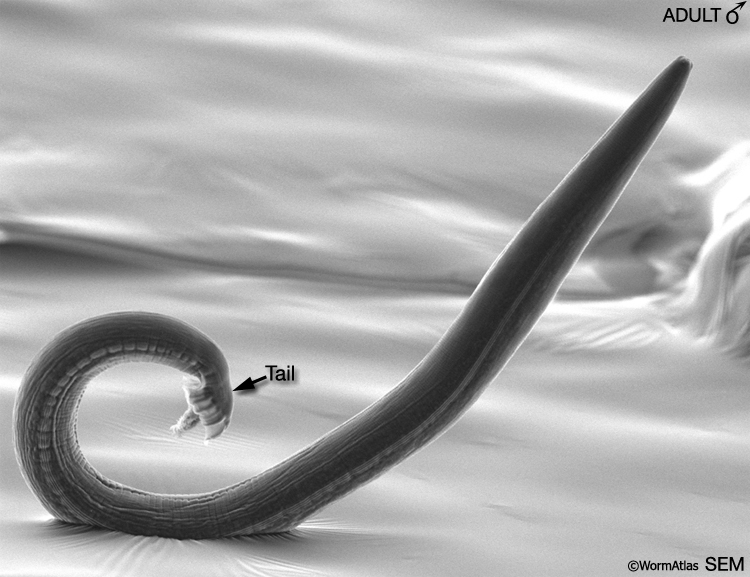
MaleIntroFIG 2A: Scanning electron microscope image of male.
MaleIntroFIG 2B: SEM image of male copulatory apparatus.
1.3 Male Behavior
Males differ from hermaphrodites in their expression of a number of behaviors including regulation of defecation (Reiner and Thomas, 1995), response to media conditioned by the same- versus opposite sex (Simon and Sternberg, 2002; White et al., 2003), mate-searching (Emmons and Lipton, 2003) and perhaps most striking of all, mating behavior (see MaleIntroFig 3; MaleIntroMOVIE 1; Wormbook-Male Mating Behavior chapter; Emmons and Sternberg, 1997). The sex-specific or sexually dimorphic cells underlying expression of some of these behaviors have been identified (Reiner and Thomas, 1995; Loer and Kenyon, 1993; Liu and Sternberg, 1995; Table 1- Emmons and Sternberg, 1997; Garcia et al., 2001).
MaleIntroFIG 3: Diagram illustrating male mating behaviors. Tail sensory structures (blue) are required for this step. (Based on Loer and Kenyon; 1993; Liu and Sternberg, 1995.)
MaleIntroMOVIE 1: Light microscopy image of a male copulating with a hermaphrodite. Click on picture to watch movie.
1.4 Generation of Male Stocks
Males (5AA; X0) arise from fusion of nullo-X gametes (gametes that lack an X chromosome) and normal X-bearing gametes. Nullo-X gametes are generated by spontaneous non-disjunction of the X chromosome during meiosis in the germ line. This, however, occurs rarely in the hermaphrodite germ line. Consequently, males occur infrequently in cultures propagated by hermaphrodite self-fertilization (ca. 0.1-0.2%; Ward and Carrel,1979; Hodgkin and Doniach, 1997). Examining male anatomy or gene expression patterns on any significant scale from such cultures is therefore impractical. Males, however, can be generated in high numbers using him mutations (high incidence of males) or by mating. him mutations increase the frequency of X-non-disjunction in the hermaphrodite germ line and consequently the number of males arising through self-fertilization (ca. 30%). him-5 and him-8 mutations are typically used as these have no apparent deleterious effects on anatomy or behavior in either sex (Hodgkin et al., 1979). Reproduction by mating produces a high frequency of males, in part, because male sperm contains an equal frequency of nullo-X and X-bearing gametes and because male-derived sperm out-compete hermaphrodite sperm in the fertilization of oocytes (Ward and Carrel, 1979). There are several methods for generating parent males (P0s) for a mating from non-him strains (1) heat-shock treatment (Sulston and Hodgkin, 1988: set up 3 plates, each containing 6 L4 stage hermaphrodites. Incubate at exactly 30°C: 1 plate for 5hrs, 1 for 5.5hrs and 1 for 6hrs); (2) Exposure to ethanol (Lyons and Hecht, 1997); (3) him-14 dsRNA feeding (Killian and Hubbard, 2001). Alternatively, P0s can be taken from him-5 or him-8 strains. Use of this approach has the added advantage of allowing him-5 or him-8 F3* homozygous lines to be recovered that will produce approximately 30% male progeny in subsequent generations without the need for crossing (*only hermaphrodites that are him homozygous can produce male off-spring by selfing and so during strain construction the first him males do not appear until the F3 generation).
1.5 Sexing C. elegans Larvae
For some studies it may be necessary to examine or recover males from mixed-sex populations before gross anatomical differences between the sexes become apparent. Sulston and Horvitz (1977) identified three criteria for distinguishing the sexes at L1 (MaleIntroFIG 4A). In the rectal epithelium, for example, B and Y cells have larger nuclei in the male (MaleIntroFIG 4B) and start dividing at late-L1. In hermaphrodites B does not divide and Y becomes the PDA neuron.
MaleIntroFIG 4A: Diagram of differences between male and hermaphrodite L1 larvae.
MaleIntroFIG 4B: Nomarski DIC pictures of the differences between male and hermaphrodite tails at the L1 larval stage.
In early L3, the male gonad takes a different path of elongation from that of the hermaphrodite (MaleIntroFIG 5A; Kimble and Hirsh, 1979; Hedgecock et al., 1987). Also from L3 onwards, sex-specific differences become apparent in the tail that can be readily detected under the stereomicroscope (MaleIntroFIG 5B). In males the white or clear area at the tail tip starts to expand anteriorly and tail becomes increasingly swollen due to cell proliferation (Sulston and Horvitz, 1977; Sulston et al., 1980). By mid L4, remodeling of the tail begins, starting with resorption of the tail tip (Sulston and Horvitz, 1977; Sulston et al., 1980; Nguyen et al., 1999).
MaleIntroFIG 5A: Sex-specific differences in gonad formation at the L3 larval stage.
MaleIntroFIG 5B: Light microscopy pictures of L3 and L4 males and hermaphrodites.
2 Anatomical Differences Between the Sexes
2.1 General Information
Males and hermaphrodites show substantial differences in their anatomy. Many structures are either sex-specific, being entirely unique to that sex (e.g. the hermaphrodite vulva, male sensory rays and fan), or are present in both sexes but have been sex-specifically modified (i.e. are sexually dimorphic, such as the tail tip, the anal depressor and sphincter muscles and some neurons). Currently, the only tissues thought to be sex-indifferent are the pharynx, excretory system and main body muscles (Sulston and Horvitz, 1977; Sulston et al., 1980; Hodgkin, 1988). Adult males and hermaphrodites also differ in somatic nuclei number (males: 1033; hermaphrodites: 959) and the proportion of these that are sexually specialized (40% of male and 30% of hermaphrodite nuclei). Additional male somatic cells arise through the division of cells, which in the hermaphrodite, either do not divide, undergo programmed cell death or produce alternative lineages (Sulston and Horvitz, 1977; Sulston et al., 1980; Sammut et al., 2015). Male and hermaphrodite germ lines also differ both in nuclei number and the type of gametes they produce (Klass et al., 1976; Kimble and Hirsh, 1979).
2.2 Developmental Origin of Sex-specific Structures
At hatching, male and hermaphrodite larvae are anatomically identical apart from a few cells (blue labels MaleIntroFIG 6). Most sex-specific tissues of the adult are formed from the descendents of blast cells (black labels MaleIntroFIG 6), that are present in both sexes but express different fates or lineages in each sex (Sulston and Horvitz, 1977; Sulston et al., 1980).
MaleIntroFIG 6: Diagram of sex-specific differences at L1. Blue labels indicate differences between sexes at hatching. Black labels indicate blast cells that give rise to sex-specific tissues. (Based on Sulston and Horvitz, 1977.)
2.2.1 Epithelial System
The epithelial system includes the hypodermis, cells of the lateral seam and the interfacial cells that line the major openings to the outside (e.g., anus, lined by the rectal epithelium) or sensory openings (socket and sheath cells; for overview see Male Epithelial System - Introduction). Major differences between the sexes are found in the posterior and the ventral body (MaleIntroFIG 7). In the male, cells of the posterior lateral seam generate the support cells (and neurons) of the sensory rays, instead of alae (described in Male Neuronal Support Cells - Rays and Male Hypodermis). hyp cells of the tail tip reorganize to form a blunt tail morphology (Male Hypodermis; for animation visit Fitch Lab website; Sulston and Horvitz, 1977; Sulston et al., 1980; Nguyen et al., 1999). The hypodermis (or lateral seam) secretes the cuticular fan in which the sensory rays are embedded. Internally, rectal epithelial cells (B, F, U, Y, K and K') divide to produce the cloaca and spicule channels of the proctodeum, replacing the simple larval rectum (see Male Epithelial System - The Proctodeum ). The B lineage also generates the support cells (and neurons) or the spicules. Ventrally, hypodermal P cells in the posterior generate support cells (and neurons) of the hook. The rectal epithelial cell Y produces the support cells (and neurons) of the PCS, which lies posterior to the hook. In hermaphrodites P cells in the mid-body make the vulval epithelium (and associated egg-laying neurons) (See Reproductive System - Egg-laying apparatus).
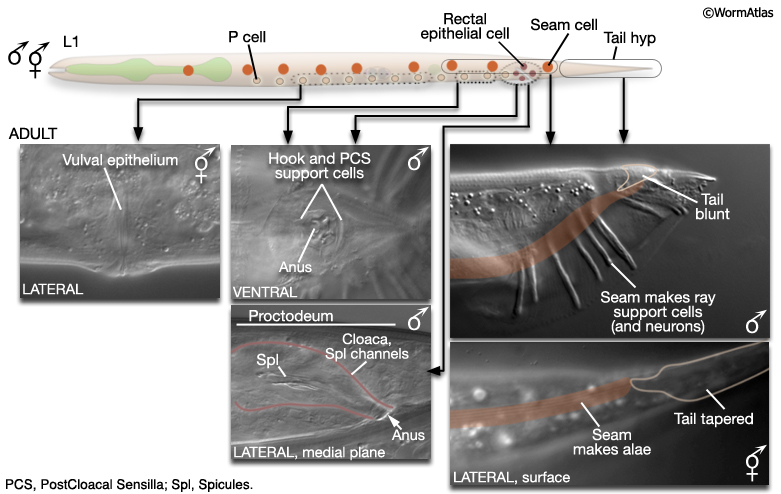
MaleIntroFIG 7: Sex-specific differences in the L1 epithelial system.
2.2.2 Nervous System
The male nervous system contains 385 neurons (91 of which are sex-specific) and the hermaphrodite 302 neurons (8 of which are sex-specific) (MaleIntroFIG 8A and 8B). Most male-specific neurons are located in the posterior (Sulston and Horvitz, 1977; Sulston et al., 1980) and many have been shown to have specific roles in male mating behavior (MaleIntroFIG 3; Table 1- Emmons and Sternberg, 1997). A smaller number of sex-specific sensory neurons and interneurons are found in the male head, and have been demonstrated to have specialized synaptic wiring in the nerve ring for pheromone detection and some forms of learning related to mate detection (White et al., 2007; Sammut et al., 2015). The hermaphrodite sex-specific neurons are located in the VNC (VC1-6) and around the vulva (HSNs) and control egg-laying behavior (see Reproductive System - Egg-laying apparatus ). There are also neurons common to both male and hermaphrodite nervous systems that exhibit sexually dimorphic synaptic patterns or morphology (e.g. BDU, PLM, and PDB; White et al., 1986; Hodgkin, 1988; Sulston et al., 1980). Potentially, more neurons of this latter class may be discovered as the nervous system of the male is reconstructed (for recent reconstructions see Individual Neurons or The Male Wiring Project).
MaleIntroFIG 8A: Sex-specific neurons at the L1 stage.
MaleIntroFIG 8B: Sex-specific neurons in adult tail regions.
2.2.3 Reproductive System
The reproductive system consists of the somatic gonad, germ line (MaleIntroFIG 9) and associated muscles (MaleIntroFIG 10) and neurons (MaleIntroFIG 8A) (see Male Somatic Gonad and Male Germ Line. In both sexes, the somatic gonad and germ line arise from the gonadal primordium (GP), which consists of 4 cells at L1, Z1-Z4 (Kimble and Hirsh, 1979). These cells execute sex-specific lineages during development establishing a distinct reproductive system in each sex. The adult male gonad consists of a single J-shaped arm that connects to the cloaca (rectal epithelial tube) of the proctodeum. The cloaca opens to the environment at the anus (cloacal opening) (for details see Male Reproductive System - Proctodeum; Sulston and Horvitz, 1977; Sulston et al., 1980; MaleIntroFIG 7). The male germ line produces only male gametes (sperm) (see Male Germ Line; Hirsh et al., 1976; Klass et al., 1976). By contrast, the adult hermaphrodite gonad consists of 2 U-shaped arms that join at the mid-body to a common uterus. The uterus opens to the exterior via the vulva. The hermaphrodite germ line produces female gametes (oocytes) and a limited number of sperm (see Hermaphrodite Reproductive System - Germ Line ).
MaleIntroFIG 9: Sex-specific differences in the reproductive system.
2.2.4 Muscle System
In this system, males and hermaphrodites differ most in their sex muscles (MaleIntroFIG 10). The sex muscles of the male are necessary for several steps in mating behavior (see Male Muscle System Overview and Male-Specific Muscles; Loer and Kenyon, 1993; Garcia et al., 2001). In hermaphrodites, the sex muscles control egg-laying behavior. In both males and hermaphrodites sex muscles are descended from M, which executes different lineages in each sex (Sulston and Horvitz, 1977; Sulston et al., 1980). In the male M generates 41 muscles (of 7 types) that are distributed throughout the posterior; in the hermaphrodite 16 sex muscles (of 2 types) are produced and these are centered on the uterus and vulva.
MaleIntroFIG 10: Sex muscles of the adult male and hermaphrodite.
2.2.5 Coelomocyte System
At hatching males and hermaphrodites have the same number of coelomocytes (ccs) but the position of two differ with respect to the gonadal primordium (see MaleIntroFIG 6). During larval development M generates additional ccs in both sexes. In the male the M lineage produces a single cc, located towards the tail; in the hermaphrodite M generates a bilateral pair of ccs, located in the dorsal posterior half of the animal (see Coelomocyte System; Sulston and Horvitz, 1977; Sulston et al., 1980).
2.2.6 Alimentary System
The alimentary system consists of the pharynx, the intestine, muscles and neurons required for defecation, the rectum and anus. In the male the simple larval rectum is replaced by the proctodeum (MaleIntroFIG 11; for details see Male Epithelial System - The Proctodeum). This structure is a common outlet for both the alimentary tract and the genital tract. It includes the non-sex-specific rectal glands and valve and several male-specific structures: the cloaca, spicule channels and spicules. These latter structures are formed by the progeny of B, F, U, K and P12. In hermaphrodites most of these cells do not divide. Muscles and neurons are also associated with the proctodeum. Some are sex-specific and while others are present in both sexes but modified in the male (the sphincter and anal depressor muscles) to accommodate the dual role of the proctodeum in defecation and ejaculation (Sulston and Horvitz, 1977; Sulston et al., 1980). In the hermaphrodite, but not the male, the intestine produces yolk protein from L4 onwards, which is taken up by developing oocytes in the gonad.
MaleIntroFIG 11: Sex-specific differences in the alimentary system.
4 References
Anderson, P. 1995. Mutagenesis. Methods Cell Biol. 48: 31-58. Abstract
Cassada, R.C. and Russell, R.L. 1975. The dauer larva, a postembryonic developmental variant of the nematode Caenorhabditis elegans. Dev. Biol. 46: 326-342. Abstract
de Bono, M. 2003. Molecular approaches to aggregation behavior and social attachment. J. Neurobiol. 54: 78-92. Article
Emmons, S.W. and Lipton, J. 2003. Genetic basis of male sexual behavior. J Neurobiol. 54: 93-110. Article
Emmons S.W. and Sternberg, P.W. 1997. Male Development and Mating Behavior. In C. elegans II (ed. D. L. Riddle et al.). Chapter 12. pp.295-334. Cold Spring Harbor Laboratory Press, Cold Spring Harbor, New York. Article
Garcia, L.R., Mehta, P. and Sternberg, P.W. 2001. Regulation of distinct muscle behaviors controls the C. elegans male's copulatory spicules during mating. Cell 107: 777-788. Article
Hall, D.H. and Russell, G.J. 1991. The posterior nervous system of the nematode Caenorhabditis elegans: Serial reconstruction of identified neurons and complete pattern of synaptic interactions. J. Neurosci. 11: 1-22. Article
Hedgecock, E.M., Culotti, J.G., Hall, D.H. and Stern, B.D. 1987. Genetics of cell and axon migrations in Caenorhabditis elegans. Development 100: 365-382. Abstract
Hirsh, D., Oppenheim, D. and Klass, M. 1976. Development of the reproductive system of Caenorhabditis elegans. Dev. Biol. 49: 200-219. Abstract
Hodgkin, J. 1988. Sexual dimorphism and sex determination. In The nematode C. elegans (ed. W.B. Wood). Chap. 9. pp 243-279. Cold Spring Harbor Laboratory Press, Cold Spring Harbor, New York. Abstract
Hodgkin, J. and Doniach, T. 1997. Natural variation and copulatory plug formation in Caenorhabditis elegans. Genetics 146: 149-164. Article
Hodgkin, J., Horvitz, H.R. and Brenner, S. 1979. Nondisjunction mutants of the nematode C. elegans. Genetics 91: 67-94. Article
Jorgensen, E.M. and Mango, S.E. 2002. The art and design of genetics screens: Caenorhabditis elegans. Nat. Rev. Genet. 3: 356-369. Abstract
Killian, D.J. and Hubbard, E.J. 2004. C. elegans pro-1 activity is required for soma/germline interactions that influence proliferation and differentiation in the germ line. Development 131: 1267-1278. Article
Kimble, J. and Hirsh, D. 1979. The postembryonic cell lineages of the hermaphrodite and male gonads in Caenorhabditis elegans. Dev. Biol. 70: 396-417. Article
Klass, M., Wolf, N. and Hirsh, D. 1976. Development of the male reproductive system and sexual transformation in the nematode C. elegans. Dev. Biol. 52: 1-18. Abstract
Liu, K.S. and Sternberg, P.W. 1995. Sensory regulation of male mating behavior in Caenorhabditis elegans. Neuron 14: 79-89. Article
Loer, C.M. and Kenyon, C.J. 1993. Serotonin-deficient mutants and male mating-behavior in the nematode Caenorhabditis elegans. J. Neurosci. 13: 5407-17. Article
Lyons, L.C. and Hecht, R.M. 1997. Acute ethanol exposure induces nondisjunction of hte X chromosome during spermatogenesis. Worm Breeders Gazette 14: 52. Article
Nguyen, C.Q., Hall, D.H., Yang, Y. and Fitch, D.H.A. 1999. Morphogenesis of the Caenorhabditis elegans male tail tip. Dev. Biol. 207: 86-106. Article
Rankin, C.H. 2002. From gene to identified neuron to behaviour in Caenorhabditis elegans. Nat. Rev. Genet. 3: 622-630. Abstract
Reiner, D.J. and Thomas, J.H. 1995. Reversal of a muscle response to GABA during C. elegans male development. J. Neurosci. 15: 6094-6102. Article
Sammut, M., Cook, S.J., Nguyen, K., Felton, T., Hall, D.H., Emmons, S.W., Poole, R.J. and Barrios, A. 2015.
Glia-derived neurons are required for sex-specific learning in C. elegans. Nature, 526, 385-390. doi:10. 1038/nature15700 Article
Simon, J.M. and Sternberg, P.W. 2002. Evidence of a mate-finding cue in the hermaphrodite Caenorhabditis elegans. Proc. Nat. Acad. Sci. 99: 1598-1603. Article
Sulston, J.E. 1976. Post-embryonic development in the ventral cord of Caenorhabditis elegans. Philos. Trans. R. Soc. Lond. B Biol. Sci. 275: 287-297. Article
Sulston, J. 1988. Cell lineage. In The nematode C. elegans (ed. W.B. Wood). Chapter 5. pp 123-155. Cold Spring Harbor Laboratory Press, Cold Spring Harbor, New York. Abstract
Sulston, J. and Hodgkin, J. 1988. Methods. In The nematode C. elegans (ed. W.B. Wood). pp v 587-606. Cold Spring Harbor Laboratory Press, Cold Spring Harbor, New York. Abstract
Sulston, J.E. and Horvitz, H. R. 1977. Post-embryonic cell lineages of the nematode Caenorhabditis elegans. Dev. Biol. 56: 110-156. Article
Sulston, J.E., Albertson, D.G. and Thomson, J.N. 1980. The Caenorhabditis elegans male: Postembryonic development of nongonadal structures. Dev Biol. 78: 542-576. Article
Sulston, J.E., Schierenberg, E., White J.G. and Thomson, J.N. 1983. The embryonic cell lineage of the nematode Caenorhabditis elegans. Dev. Biol. 100: 64-119. Article
Ward, S. and Carrel, J.S. 1979. Fertilization and sperm competition in the nematode C. elegans. Dev. Biol. 73: 304-321. Abstract
White, J. 1988. The Anatomy. In The nematode C. elegans (ed. W.B. Wood). Chapter 4. pp 81-122. Cold Spring Harbor Laboratory Press, Cold Spring Harbor, New York. Abstract
White J.G., Southgate, E., Thomson, J.N. and Brenner, S. 1986. The structure of the nervous system of the nematode C. elegans. Philos. Trans. R. Soc. Lond. Series B. Biol. Sci. 314: 1-340. Article
White, J.G., Albertson, D.G. and Anness, M.A.R. 1978. Connectivity changes in a class of motoneurone during the development of a nematode. Nature 271: 764-766. Abstract
White, J.Q., Nicholas, T.J., Gritton, J., Truong, L., Davidson, E.R. and Jorgensen, E.M. 2007. The sensory circuitry for sexual attraction in C. elegans males. Curr. Biol. 17: 1847-57. Article
|